Abstract
Adipose stem cells (ASCs) have shown therapeutic promise for various conditions, including burn injury. While ASCs have immunomodulatory properties, concerns exist over pro-coagulant activity after intravenous (IV) administration. In the present study, we examined IV human ASC delivery in terms of coagulation, organ function, and inflammation in a 40% total body surface area (TBSA) swine burn model. Anesthetized female Yorkshire swine were burned and randomized to receive 15 ml/kg Lactated Ringer’s containing: no ASCs; a low dose (5 × 105 ASCs/kg); or a high dose (5 × 106 ASCs/kg). For biochemical analysis, blood was collected at baseline (BL), 3, 6, 12, and 24 h post-burn, while kidney and liver tissue was collected post-euthanasia. A significant, but transient, effect of ASCs was seen on prothrombin times and INR, wherein low doses revealed slight hypercoagulation. Burns increased partial thromboplastin time, fibrinogen, and d-dimer levels, which was unchanged with ASC administration. ASCs tended to exacerbate increases in bilirubin at 3 h, but this didn’t reach statistical significance. A significant effect of ASCs on creatinine and BUN was seen, wherein low doses elevated levels at 24 h (creatinine, P = 0.0012; BUN, P = 0.0195). Hepatic and renal TUNEL staining were similar for all groups. A dose-dependent decrease in IL-8 was observed, while low doses significantly increased IL-1RA at 3h (P = 0.050), IL-12 at 12h (P = 0.021) and IL-6 at 24 h post-burn (P = 0.035). IV administration of xenogeneic ASCs slightly increased coagulation, but effects on burn-induced renal and hepatic dysfunction effects were minimal. Despite some significant immunomodulation, organ dysfunction effects were modest. Collectively, this study provides evidence to be skeptical about xenogeneic ASC administration in regards to burns.
Worldwide, burns account for 180,000 deaths annually with nearly 11 million people requiring medical attention for burn injuries.1 Large total body surface area (TBSA) burns lead to significant pathophysiologic changes including inflammation, endotheliopathy, and fluid shifts. As a consequence of these aberrations, patients are at an increased risk of death and a high risk of developing multi-organ dysfunction (MOD) such as acute kidney injury (AKI) or acute liver injury (ALI).2–4
AKI due to burn injury may be classified as early or late AKI (cutoff typically ~5 days), with early AKI predictive of mortality.5 In the context of burn, severity of AKI increases as the severity of burn injury increases.3 Burn-induced AKI is mediated, in part, by inflammation,6 with IL-6 and IL-8 levels associated with higher mortality in patients with renal dysfunction.7 Similarly, ALI is a complication of severe burns in both human patients and animal models of burn injury.8–11 Mechanistically, hepatocyte apoptosis and inflammation have been observed postburn injury in rodents.12–14 In large TBSA burn patients, persistent increases in liver weight are seen and bilirubin levels are a major prognostic factor for mortality risk.10,11,15,16 Taken together, strategies to target multiple components of MOD such as AKI and ALI would mitigate burn shock, benefit burn patients, and improve outcomes.
To this end, strategies including mesenchymal stem cells (MSCs) have become attractive due to the multipotent and immunomodulatory abilities of these cells.17,18 When administered intravenously, MSCs can home to sites of injury and have been detected in various tissues without ectopic tissue formation or long term engraftment in the host.19–21 MSCs are available from a variety of anatomical locations, and have been isolated from numerous sources such as lipoaspirates (termed adipose stem cells: ASCs), and also from debrided burned tissue which is often discarded as medical waste.22 ASCs in particular have been shown to possess enhanced immunomodulatory abilities compared to MSCs from other tissues,23,24 which may confer a greater potential to prevent AKI or ALI. To date, the majority of studies using ASCs for burn injury have focused on ensuing effects on wound healing25,26 even when the ASCs are delivered in an intravenous (IV) fashion.27,28 One impediment to employing the IV delivery of ASCs has been over concerns relating to their thrombogenic potential. While IV delivery of ASCs has been examined in animal models of inhalation injury,29 there is less evidence on their efficacy in reducing burn-induced organ dysfunction.
To combat some of the challenges inherent in cell expansion, the prospect of xenotransplantation of MSCs could provide an off-the-shelf alternative. Considering use in animal models, xenotransplantation of human ASCs has been shown to decrease alanine transaminase (ALT) and aspartate aminotransferase (AST) in transplant-related ALI via mitigation of inflammation30 and reductions in hepatic apoptosis.31,32 Moreover, human ASC administration has reduced serum creatinine and BUN, as well as renal apoptosis and structural damage which was associated with lower inflammatory cytokine production.33 While xenogeneic administration of ASCs has been shown to reduce inflammation and improve ALI and AKI, their potential efficacy in burn-induced MOD is far less studied. To this end, we hypothesized that IV injection of two doses of human ASCs would improve burn-induced AKI and ALI by reducing inflammation in a 40% TBSA burn model in pigs.
MATERIALS AND METHODS
This research was conducted in compliance with the Animal Welfare Act, the implementing Animal Welfare Regulations, and the principles of the Guide for the Care and Use of Laboratory Animals, National Research Council. The facility’s institutional animal care and use committee approved all research conducted in this study. The facility where this research was conducted is fully accredited by the Association for Assessment and Accreditation of Laboratory Animal Care. Eighteen (n = 18) female, sexually immature Yorkshire pigs (39.9 ± 0.6 kg) were used in this study. Upon arrival to our institute, animals had a minimum 7-day acclimation period during which they were singly housed, with ad libitum access to water, and fed a commercial laboratory pig formulated pelleted diet. Randomization to different groups was performed so that each shipment of animals was represented evenly amongst the groups.
Culturing of Human Adipose Derived Stem Cells
Human adipose derived stem cells (hASCs) were purchased from Rooster Bio (Rooster Bio Incorporated, Frederick, MD), with associated media prepared according to the manufacturer’s instructions. Two vials of 1 × 106 or 1 × 107 hASCs were thawed, washed, and cultured at 37°C, 5% CO2 for animals receiving low and high doses of ASCs, respectively. In this respect, animals were randomized before the day of the experiment to allow for preparation of ASCs.
Thermal Injury
Administration of burn wounds and postoperative animal care were performed as previously described.34–38 The timeline for the experiment described here is given in Supplemental Figure 1. Anesthesia was induced with Telazol (6 mg/kg) and followed by 1%–3% isoflurane. Indwelling catheters were placed in the external jugulars by standard cut down procedures and lines were tunneled subcutaneously to exit the back of the neck. Prior to the burn injury, analgesia was achieved with intramuscular Buprenex-HCl Sustained Release (0.1–0.24 mg/kg, Veterinary Technologies/ZooPharm, Windsor, CO). After the animal was catheterized, a contact burn was created using brass probes (9 cm × 15 cm blocks or 5 cm × 5 cm blocks) heated to 100°C contacting the skin for 25–35 s to achieve a full thickness burn under anesthesia.39 This was repeated until 40% total body surface area was burned including the dorsum, flanks, and hind limbs. Animals were allowed to recover in metabolic cages to monitor fluid intake. Twice a day postburn, the animals were given access to 15 ml/kg World Health Organization’s oral rehydration salt solution (WHO-ORS) containing 5-chloro-2′-deoxyuridine (CldU) at a concentration of 1 mg/ml to allow for labeling of endogenous proliferating cells.
Infusion of Human Adipose Derived Stem Cells
After recovery in the metabolic cage, animals were resuscitated in a bolus fashion with Lactated Ringer’s solution (LR) at a volume of 15 ml/kg containing one of the three ASC doses: 1) no ASCs (none, n = 6); 2) 5 × 105 ASCs/kg (low, n = 6); or 3) 5 × 106 ASCs/kg (high, n = 6). ASCs were trypsinized on day 5 of culture, and centrifuged at 200g for 10 min at room temperature. ASC viability was determined via Trypan blue staining and then washed in 50 ml of LR followed by another centrifugation at 200g for 10 min at room temperature. The cell pellet was resuspended in 50 ml of LR and injected into a 1 liter bag of LR for a final concentration of 3.3 × 107 ASCs/ml or 3.3 × 108 ASCs/ml: fluid was administered over the course of 15 min.
Blood and Urine Analysis
Blood was collected at baseline (0 h, pre-burn), 3 h (post-fluids), 6 h, 12 h, and 24 h postburn. Venous blood was collected in: sodium citrate tubes for coagulation parameters Prothrombin time (PT), International normalized ratio (INR), partial thromboplastin time (PTT), fibrinogen concentration (FIB), and D-dimer (DDi.L) concentrations run on a Siemens BCS® XP System; lithium-heparin containing tubes for biochemical analysis with a Siemens Dimension® EXL™ 200 Integrated Chemistry System; and K2 EDTA tubes for complete blood cell analysis with a Siemens ADVIA® 120 System. For blood gas analysis, blood was loaded into an iSTAT CG4+ cartridge (Abbott Point of Care, Princeton, NJ).
Histological Analysis
After euthanasia at 24 h, kidney and liver tissue was flash frozen in optimal cutting temperature compound and sectioned into 5 μm slices. Terminal deoxynucleotidyl transferase dUTP nick-end labeling (TUNEL, Roche Cat # 11684809910) staining was performed according to the manufacturer’s recommendations, and slides were counterstained with mounting medium containing 4′,6-diamidino-2-phenylindole (DAPI, Vector Labs. Cat H-1200). Sections were also immunostained with a 1:100 dilution of anti-5-bromo-2′-deoxyuridine antibody (Thermo Fisher Scientific, Cat # B35130, which cross-reacts with 5-chloro-2′-deoxyuridine) followed by appropriate secondary antibody labeling and mounting media containing DAPI. Whole renal and hepatic sections were imaged using an AxioScanZ1 slide scanner (Carl Zeiss, Thornwood, NY) and put through automated quantification of colors with ImageJ software version 1.51 (Bethesda, MD). High-magnification images were taken of kidney and liver samples from each animal to analyze TUNEL staining and separated into red, green, and blue channels for the quantification of channel intensities, with apoptosis reported as the mean intensity of the green channel.
Western Blotting
One gram of kidney and liver tissue collected at 24 h postburn was homogenized in mammalian protein extraction buffer containing protease phosphatase inhibitor (Sigma–Aldrich; St. Louis, MO). Protein concentration was quantified via bicinchoninic acid protein assay kit according to the manufacturer’s instructions (Thermo Scientific; Waltham, MA). Precast polyacrylamide electrophoresis gels were loaded with 50ug of protein per lane (Bio-Rad; Hercules, CA) and then transferred to a nitrocellulose membrane (Bio-Rad; Hercules, CA). After an hour of blocking the membrane in 5% nonfat dry milk solution containing 0.1% Tween-20, the membrane was incubated with 1:1,000 of either Caspase-3 antibody (Abcam; Cambridge, UK) for three days or β-actin antibody (Cell Signaling Technology; Danvers, MA) overnight at 4°C. The membrane was incubated at room temperature with 1:10,000 of IRDye antibodies (LI-COR; Lincoln, NE) for 1 h in the dark followed by fluorescent detection captured in both 700- and 800-nm channels in a single scan using LI-COR Odyssey (LI-COR; Lincoln, NE). Band intensity was quantified using Image Studio Software, version 2.1.15 (LI-COR; Lincoln, NE).
Measurement of Cytokines/Chemokines in Porcine Plasma Samples
At the aforementioned time points, blood was collected into K2 EDTA-containing tubes and centrifuged at 1,000g for 10 min, aliquoted, and stored at −80˚C until analysis. For detection of cytokines and chemokines in the plasma, the Milliplex MAP Porcine Cytokine/Chemokine Magnetic Bead Panel (EMD Millipore, Billerica, MA) was used according to the manufacturer’s instructions. Each sample was run in duplicate.
Statistical Analysis
Statistical analysis was expressed as the mean ± SEM, and P < 0.05 was considered significant. Repeated measures data was analyzed via GraphPad Prism using 2-way ANOVA and Tukey’s test for multiple comparisons to determine the significance of dosage of cells and time. Data on terminal tissues (eg, Westerns) was tested for normality using Pearson’s omnibus normality test, and subsequently analyzed with one-way ANOVA or Kruskal–Wallis testing as appropriate.
RESULTS
Slight Hypercoagulation After Low Doses of ASCs
To address concerns over potential thrombogenic events of IV infusion of ASCs, we performed coagulation assays. Two-way ANOVA revealed a significant effect of ASCs on Prothrombin time (PT) (Figure 1A), in which the low dose of cells exacerbated the transient decrease in PT (time effect, P = 0.0049; ASC effect, P = 0.0047). Similar reductions in International normalized ratio (INR) (Figure 1B) were seen; however only the effect of cells attained significance (P = 0.0030), again with the low dose of cells reducing INR. Alternatively, burn-induced increases in partial thromboplastin time (PTT) (Figure 1C), fibrinogen (FIB) (Figure 1D), D-dimers (DDi.L) (Figure 1E), and platelets (Figure 1F) were not affected by infusion of ASCs. Still, a significant effect of burn was observed for these parameters (PTT, time P = 0.0002; FIB, time P < 0.0001; D-dimers, time P < 0.0001; platelets, time P = 0.0002).
Figure 1.
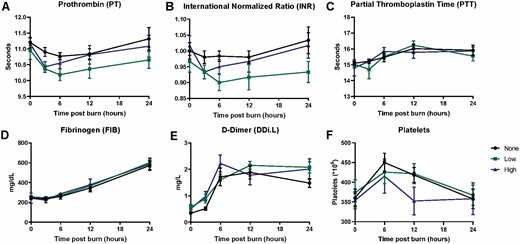
Absence of global hypercoagulation after infusion of human ASCs postburn. A significant overall effect of ASCs was seen on PT (A, P = 0.0047) and INR (B, P = 0.0030), which was not true for PTT (C), Fibrinogen (D), or D-dimer (E). A significant effect of burns was seen for all readouts except for PTT (P ≤ 0.0002 for each) in this study. Data points are represented as the mean ± SEM.
Figure 1.
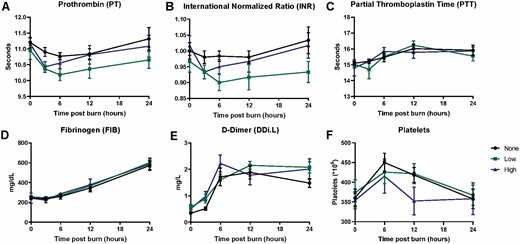
Absence of global hypercoagulation after infusion of human ASCs postburn. A significant overall effect of ASCs was seen on PT (A, P = 0.0047) and INR (B, P = 0.0030), which was not true for PTT (C), Fibrinogen (D), or D-dimer (E). A significant effect of burns was seen for all readouts except for PTT (P ≤ 0.0002 for each) in this study. Data points are represented as the mean ± SEM.
Effect of ASCs on Acute Liver Injury Markers Postburn
We examined liver biomarkers to address ASC effects on burn-induced ALI (Figure 2A). Levels of total bilirubin transiently increased due to burn injury (P < 0.0001) reaching a peak at 3–6 h. Infusion of ASCs tended to exacerbate this increase in bilirubin, but this did not reach statistical significance (3 h postburn, no cell vs high dose, P = 0.078) (Figure 2A). ALT steadily increased in all treatments groups peaking at 24 h postburn (114.67 ± 7.21 U/l for no cell group, 109.17 ± 10.54 U/l for low dose group, and 100.17 ± 7.4 U/l for high dose group). While infusion of a high dose of ASCs tended to decrease ALT levels postburn, this trend compared to the no cell group did not reach statistical significance (overall effect of ASCs, P = 0.057). Similarly, AST levels steadily increased due to burn injury from 3 h postburn to 6 h postburn (P < 0.0001) for all treatment groups, but there was no significant effect of ASCs (P = 0.236). From this time point, AST levels gradually decreased but did not reach baseline levels for any group (Figure 2A).
Figure 2.
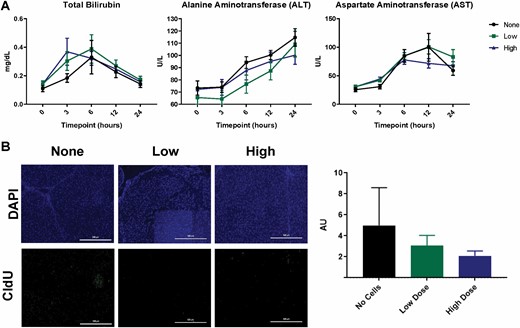
Liver injury and proliferation. Total bilirubin, alanine aminotransferase (ALT), and aspartate aminotransferase (AST) (A). A significant effect of time was observed for the total bilirubin (A) (P < 0.0001), ALT (P < 0.0001), and AST (P < 0.0001). Detection of CldU in liver sections via immunohistochemistry (B) revealed a slight reduction of proliferation in hepatocytes due to ASCs, which was not significant upon quantification. Data points are represented as the mean ± SEM.
Figure 2.
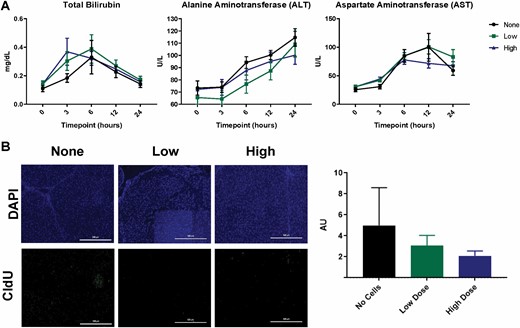
Liver injury and proliferation. Total bilirubin, alanine aminotransferase (ALT), and aspartate aminotransferase (AST) (A). A significant effect of time was observed for the total bilirubin (A) (P < 0.0001), ALT (P < 0.0001), and AST (P < 0.0001). Detection of CldU in liver sections via immunohistochemistry (B) revealed a slight reduction of proliferation in hepatocytes due to ASCs, which was not significant upon quantification. Data points are represented as the mean ± SEM.
Effect of ASC Infusion on Hepatocyte Proliferation and Apoptosis
We labeled endogenously proliferating cells within the liver with antibodies to CldU (Figure 2B). A dose-dependent reduction in cell proliferation in the liver at 24 h postburn was observed for animals receiving ASCs, but this did not reach statistical significance (P = 0.1865) (Figure 2B). To assess the level of hepatic apoptosis postburn, Western blotting for Caspase-3 and TUNEL staining of livers 24 h postburn was performed (Figure 3). Caspase-3 expression was significantly increased at 24 h postburn for animals receiving a low dose of ASCs relative to the no cell group (no cell vs low dose, P = 0.0249) (Figure 3A). TUNEL staining of the livers shows the widespread localization of apoptotic hepatocytes (Figure 3B), but was not different amongst the groups (P = 0.3666). Taken together, the effect of ASCs on hepatocyte turnover is, if anything, detrimental.
Figure 3.
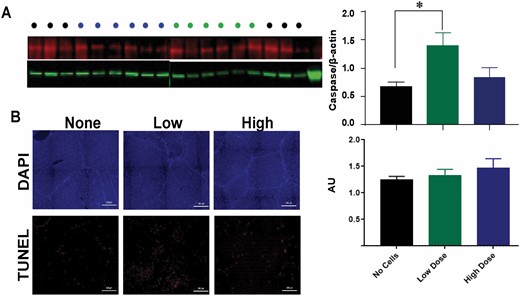
Apoptosis in the liver. Caspase-3 levels in liver lysates via Western blot (A) and TUNEL staining in liver sections via immunohistochemistry (B) were quantified for each treatment group detected at 24 h postburn. Data points for A and B are represented as the mean ± SEM. * Low dose significantly higher than no dose (P < 0.05).
Figure 3.
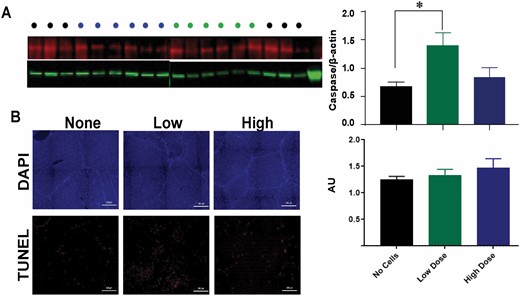
Apoptosis in the liver. Caspase-3 levels in liver lysates via Western blot (A) and TUNEL staining in liver sections via immunohistochemistry (B) were quantified for each treatment group detected at 24 h postburn. Data points for A and B are represented as the mean ± SEM. * Low dose significantly higher than no dose (P < 0.05).
Effect of ASCs on Acute Kidney Injury Markers Postburn
We examined urine output and renal biomarkers to address ASC effects on burn-induced AKI (Figure 4A). Total urine output for the experiment decreased in a dose-dependent manner for the ASC groups relative to the no cell group, but was not statistically significant (P = 0.4561). A significant effect of cells was observed for plasma creatinine (P = 0.0012), wherein the low dose of cells increased creatinine levels which was statistically significant at 24 h postburn (no cell vs low dose, 1.57 ± 0.26 vs 2.81 ± 0.76, P = 0.0023; low dose vs high dose, 2.81 ± 0.76 vs 1.33 ± 0.12, P = 0.0002). A significant effect of time and cells was observed for plasma BUN levels (time, P < 0.0001; cells, P = 0.0195). For all treatment groups, BUN levels steadily increased from baseline to 12 h postburn, and a statistically significant increase in BUN levels was observed for the low dose group compared to the high dose group at 24 h postburn (low dose vs high dose, 23.72 ± 4.28 vs 16.08 ± 2.20; P = 0.0149) (Figure 4A).
Figure 4.
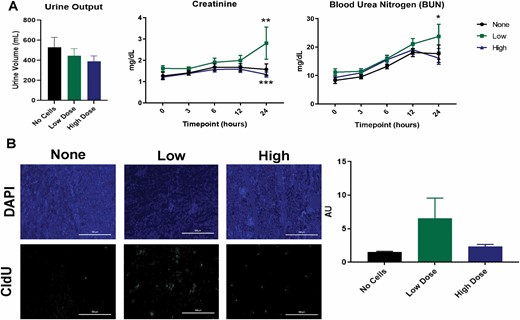
Kidney injury and proliferation. Measurement of total urine volume, creatinine, and blood urea nitrogen (BUN) levels (A). Low ASC doses led to increases in circulating renal function biomarkers (*P < 0.05, **P < 0.01, ***P < 0.001). Detection of CldU in kidney sections via immunohistochemistry and the mean CldU in kidney sections (B) for each treatment group (n=6 per group) were conducted at 24 h postburn. Data points are presented as mean ± SEM for (A) and (B).
Figure 4.
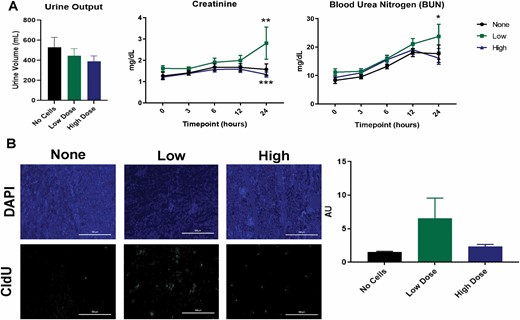
Kidney injury and proliferation. Measurement of total urine volume, creatinine, and blood urea nitrogen (BUN) levels (A). Low ASC doses led to increases in circulating renal function biomarkers (*P < 0.05, **P < 0.01, ***P < 0.001). Detection of CldU in kidney sections via immunohistochemistry and the mean CldU in kidney sections (B) for each treatment group (n=6 per group) were conducted at 24 h postburn. Data points are presented as mean ± SEM for (A) and (B).
Effect of ASC Infusion on Renal Proliferation and Apoptosis
We labeled endogenously proliferating cells within the kidney with antibodies to CldU (Figure 4B). An apparent increase in proliferating cells within the kidney was seen: however, upon quantification this was not statistically significant (P = 0.0876) (Figure 4B). Caspase-3 levels and TUNEL staining were used as a measure of the extent of apoptosis in the kidney postburn. No difference was seen in Caspase-3 expression between the treatment groups (P = 0.4074) (Figure 5A). The amount of TUNEL positive cells in the kidney (Figure 5B) tended to decrease in a dose-dependent manner, however this also did not reach significance (P = 0.3196). Despite similar levels of caspase-3 expression, ASC infusion resulted in a non-significant reduction of apoptosis in the kidney.
Figure 5.
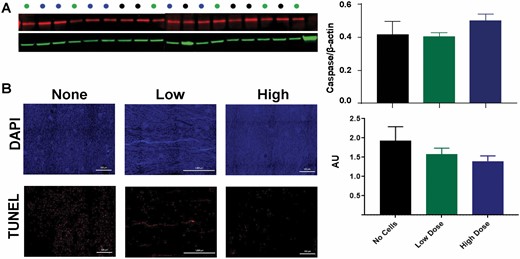
Apoptosis in the kidney. Caspase-3 levels in kidney lysates via Western blot (A) and TUNEL staining in kidney tissue slices via immunohistochemistry (B) were quantified for each treatment group detected at 24 hours postburn, but did not reveal any significant differences. Data points for (A) and (B) are presented as mean ± SEM.
Figure 5.
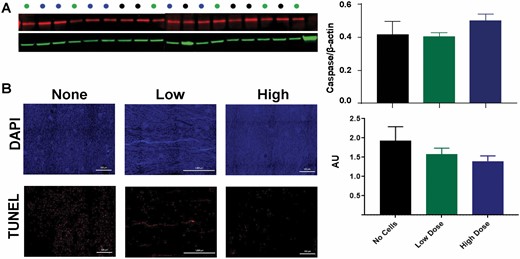
Apoptosis in the kidney. Caspase-3 levels in kidney lysates via Western blot (A) and TUNEL staining in kidney tissue slices via immunohistochemistry (B) were quantified for each treatment group detected at 24 hours postburn, but did not reveal any significant differences. Data points for (A) and (B) are presented as mean ± SEM.
Immunomodulatory Effects of ASCs
To address potential immunomodulatory effects of ASC infusion, we examined the levels of common cytokines IL-1RA, IL-12, IL-6, and IL-8 (Figure 6), with overall levels of circulating leukocytes given in Supplemental Table 1. We found a significant effect of cells for IL-1RA postburn (P = 0.0055), wherein burn-induced elevations in this anti-inflammatory cytokine was increased with ASC infusion, significantly so in the low dose group. IL-1RA levels remained elevated above baseline from 3 h to 24 h postburn for the low dose group (3 h postburn, P = 0.050, no cell vs. low dose). For the pro-inflammatory IL-12, a significant effect of cells and time was observed postburn (P = 0.0033 and P = 0.0003, respectively). The low dose of ASCs elevated this cytokine compared to baseline levels and compared to other groups at 6 (6 h postburn, P = 0.064, no cell vs. low dose) and 12 h, while no cells and high dose of ASCs remained low compared to baseline (12 h postburn, P = 0.021, no cell vs low dose; 12 h, P = 0.029, low dose vs high dose) (Figure 6B).
Figure 6.
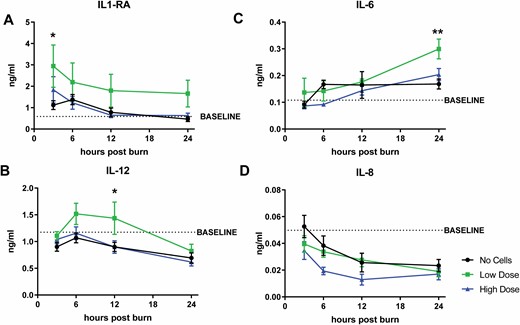
Circulating cytokine levels postburn and IV-ASCs. Levels of anti-inflammatory cytokine IL-1RA (A) and pro-inflammatory cytokines, (B) IL-12, (C) IL-6, and (D) IL-8 were significantly different due to ASC infusion. Data points are presented as mean ± SEM. An asterisk (*) indicates a significant difference between treatment groups of (P < 0.05) while (**) indicates a significant difference of mean ± SEM between treatment groups of (P < 0.01).
Figure 6.
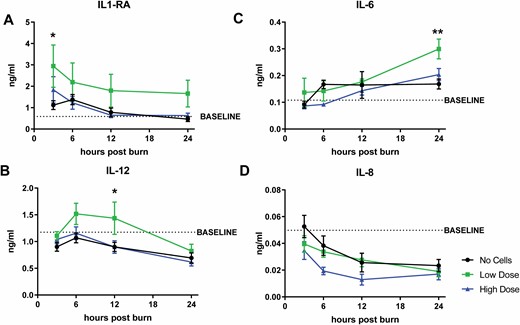
Circulating cytokine levels postburn and IV-ASCs. Levels of anti-inflammatory cytokine IL-1RA (A) and pro-inflammatory cytokines, (B) IL-12, (C) IL-6, and (D) IL-8 were significantly different due to ASC infusion. Data points are presented as mean ± SEM. An asterisk (*) indicates a significant difference between treatment groups of (P < 0.05) while (**) indicates a significant difference of mean ± SEM between treatment groups of (P < 0.01).
The often examined IL-6 also increased due to burn injury (P < 0.0001) starting at 12 h post-burn. Moreover, there was a significant effect of cells observed for IL-6 (P = 0.027), wherein the levels of IL-6 were increased after infusion of the low dose of ASCs. At 24 h, this reached significance (low dose vs no cell, 0.30 ± 0.037 ng/ml and 0.17 ± 0.019 ng/ml, respectively; P = 0.0044) which was also significantly different than the high dose group (0.20 ± 0.023 ng/ml; P = 0.035) (Figure 6C). For IL-8, burn significantly reduces circulating levels (P = 0.0002) for the entire 24 h experiment. A significant effect of ASCs was also seen (P = 0.0088), wherein ASCs decreased IL-8 levels in a dose-dependent manner compared to no cells, which was sustained longer after infusion of the high dose of ASCs (Figure 6D).
Discussion
Despite advances in burn care, extensive burns present with a complex pathophysiology that result in significant multi-organ dysfunction and mortality. Regenerative medicine approaches such as ASCs have been used to counteract deleterious effects of AKI and ALI, which are both components of burn-induced multi-organ dysfunction. In the present study, hASCs were administered intravenously in a xenogeneic fashion to swine with 40% TBSA full thickness contact burns. The salient findings include that ASC infusion resulted in a decrease in clotting times driven by the extrinsic (but not the intrinsic) coagulation pathway. Additionally, beneficial effects of ASCs on hepatic function were minimal and modest effects on renal function were, if anything, detrimental. This is despite some evidence for advantageous immunomodulation with ASC infusion and variable effects on cell turnover in the liver and the kidney.
The safety of IV ASC administration has been questioned due to concerns over thrombogenic potential but evidence remains controversial. Human and animal MSCs express variable levels of tissue factor with ASCs expressing significantly higher levels of tissue factor compared to MSCs isolated from the bone marrow.40–43 Moreover, the nuances of burn injury on coagulation status are only beginning to be elucidated.44 These concerns led us to examine potential differences in coagulation status in the context of burn and ASC infusion. A transient decrease in PT time and INR was observed post-burn, which was slightly exacerbated due to ASC infusion. However, increases in extrinsic coagulation activity was not seen, as PTT was actually prolonged due to burn injury. Similarly, fibrinogen and D-dimer levels increased post-burn but were not affected by ASCs. A possible explanation for the discrepancies between the current study and previous studies is the effect of trauma on the levels of tissue factor expression on ASCs. For example, in vitro experiments showed that citrated blood from healthy donors mixed with hASCs led to a greater decrease in TEG R time than with citrated blood from trauma patients, which may be due to existing tissue factor levels.42 Further studies would be needed to see if this is the case in burns as well.
Another consideration that could explain these differences is the dosing of ASCs chosen which has been studied in animals and patients.23,45 For example, a previous study showed IV infusion of one dose of ASCs into mice resulted in increased mortality via pulmonary blood clots, where a smaller dose did not.43 Alternatively, other studies have not shown differences in pulmonary emboli formation due to ASC doses ranging from 5 × 106 to 2.5 × 108 ASCs/kg.23 In a mouse model of pneumosepsis, IV administration of 1 × 106 hASCs led to upregulation of pro-coagulant genes and microthrombi in the lung, as well as an increase in tissue factor clotting activity that resolved at 48 h post infusion.46,47 We chose a range of 5 × 105−5 × 106 ASCs/kg which, although similar to previous studies in large animals,29 is generally lower than doses used in mice when normalized to body weight. For example, the Perlee group administered 1 × 106 ASCs, which when normalized to an average 20 g mouse, leads to 5 × 107ASCs/kg. Other factors such as rate of infusion, filtration strategy, or agitation to prevent clumping should also be taken into account when comparing studies.
One strategy that remains viable is the ability to precondition ASCs in culture before their infusion, since this has been shown to increase the therapeutic potential. For example, ASC expansion in media containing low serum increases growth factor secretion such as hepatocyte growth factor (HGF) and vascular endothelial growth factor (VEGF).48 This led to a significant decrease in BUN and creatinine in chemically-induced AKI in rats.48 Treatment of hASCs with a cocktail of VEGF-A, human fibroblast growth factor (FGF-2), human epidermal growth factor (EGF), and insulin-like growth factor (IGF-1) in culture led to a significant decrease in liver fibrosis and circulating hepatic injury markers, increased proliferation of hepatocytes, and significant increase in HGF level production post liver injury.49 Exposure of MSCs to a hypoxic environment has also been shown to ameliorate AKI by decreasing renal apoptosis and tubular injury scores. Preconditioning ASCs may confer a therapeutic benefit, which was not examined in the current study. However, if this was performed in an autologous fashion, there would still be a considerable amount of time needed for ASC expansion ex vivo.
Another consideration is the route of administration of ASCs, with direct parenchymal injection being a viable option. In a rat model of kidney ischemia and reperfusion injury, hASC injection into the parenchyma of the left kidney at the time of reperfusion led to a significant increase in cell proliferation and reduction of apoptosis and renal damage at 72 h post injury,50 while maintaining immunomodulatory effects. In a porcine model of acute liver injury, intra-portal vein administration of hBM-MSCs significantly increased survival up to 6 months post injury while hepatic injury marker levels were reduced.51 In another large animal model, intra-portal administration of porcine BM-MSCs significantly prolonged the survival of pigs post injury relative to pigs receiving porcine BM-MSCs via the peripheral vein, arteria hepatica, and directly into the liver.52 Since burn patients already receive copious amounts of IV fluids, we chose to concentrate on this route of administration in order to reveal if LR would be a suitable delivery vehicle.
In this regard, our resuscitation strategy in this protocol provided a relatively low amount of fluids compared to what expected requirements would need. As such, ASC delivery occurred as a bolus, as opposed to a continuous infusion. We cannot rule out that prolonged infusion of ASCs would not either reveal more beneficial effects on organ function or result in increased thromboembolic events. The effect of ASCs on bilirubin appeared to be substantial at the 3 h time point (ie, right after infusion of the ASCs) and resolution of these elevated levels to baseline may have happened more expeditiously if infusion were to have continued. However, this would have been logistically challenging in the current protocol due to the conscious state of the animals.
Since burn injury is marked by a unique cytokine profile and both ALI and AKI are mediated by inflammation, we hypothesized that ASCs would have exerted a beneficial effect via anti-inflammatory properties. Significant increases in IL-6 and IL-8 were observed in patients early postburn with a weak positive relationship existing between TBSA, IL-6, and IL-8.53–55 In the present study, an increase in anti-inflammatory cytokine IL-1RA and pro-inflammatory IL-12 and IL-6 in pigs postburn was observed, where a significant increase was observed post infusion with a low dose of ASCs. Treatment with ASCs led to dose-dependent decreases in IL-8, though this did not reach statistical significance. In short, the modest differences seen with AKI exacerbation due to low doses of ASCs are in line with the slight increase in cytokines IL-6 and IL-12. ASC-associated reductions in IL-8 may result in alterations in neutrophil chemotaxis. It is well known that ASCs exert their effects largely through paracrine mechanisms, and pertinent humoral factors in the current study were not revealed. IL-1RA, upregulated upon MSC stimulation with pro-inflammatory cytokines,56 has also been shown to have an effect on anti-inflammatory capabilities of ASCs. Infusion of IL-1RA knockout MSCs into arthritic mice led to an exacerbation of arthritis pathology, an increase in splenic activated T-cells and reduction of anti-inflammatory T-cells, and an increase in serum IL-1beta, IL-6 and TNF-α, highlighting the importance of IL-1RA to the anti-inflammatory properties of MSCs.56,57 In this regard, we cannot rule out the reciprocal actions of burn injury and ASC infusion when it comes to inflammation.
When compared to MSCs from other tissue sources, ASCs have advantages in that they can be isolated at greater numbers and by minimally invasive procedures. Moreover, it has been demonstrated that ASCs can also be isolated from the debrided tissue during burn excision, which is often treated as medical waste.22,58,59 These cells are often expanded for use in wound healing by, for example, incorporation into tissue engineering strategies.60 To combat the time needed for ASC expansion, an off-the-shelf cell source for treating acute systemic inflammation would be attractive. To this end, we aimed at employing a xenogeneic strategy, and it may be possible that cross-species differences counteract beneficial immunomodulatory effects when infused. Our results indicate this may be the case, and that the potential for using porcine ASCs as an off-the-shelf capability in the clinical situation may not be advantageous, and may be better filled with an allogeneic strategy.
There are several limitations to this study worth mentioning. First, a limited sample size may have precluded uncovering significant effects of ASCs in several parameters. For example, ASCs appeared to have a benefit in liver functional tests (ie, bilirubin, ALT) as well as both hepatic and renal proliferation, which approached statistical significance. Considerations related to the COVID-19 pandemic and financial considerations not only precluded additional subjects, but also hindered the ability to select multiple time points for examining histological and molecular analyses. It would have been advantageous to collect tissue immediately after infusion to determine the fate of ASCs that were infused. As mentioned earlier, pretreatment of cells may have enhanced their therapeutic effects, which was also not examined in the existing study. Lastly, only one age, strain, and gender of animals were used, which would have also outstretched available resources.
Conclusions
Taken together, our results show that IV infusion of ASCs only induces a modest change in coagulation status and in both renal and hepatic function post-burn. Bolus infusion of xenogeneic ASCs would therefore be better replaced clinically by continuous infusions, or potentially allogeneic or autologous use of human ASCs. While we were surprised to see a lack of effect of the commercially available cells, this study lends insight into a field that requires further detailed investigation for therapeutic use in burn injuries.
Conflict of interest statement. The authors declare no conflicts of interest with respect to publication of this article.
The opinions or assertions contained herein are the private views of the author and are not to be construed as official or as reflecting the views of the Department of the Army, Uniformed Services University of the Health Sciences, the U.S. Government, the Department of the Army or the Department of Defense.
Funding: This work was supported by a research grant provided by the United States Army Medical Research and Development Command (USAMRDC) (Award # C_066_2017).
References
Rakkolainen
I
,
Lindbohm
JV
,
Vuola
J
.
Factors associated with acute kidney injury in the Helsinki Burn Centre in 2006−2015
.
Scand J Trauma Resusc Emerg Med
2018
;
26
:
105
.
Clark
AT
,
Li
X
,
Kulangara
R
et al.
Acute kidney injury after burn: a cohort study from the parkland burn intensive care unit
.
J Burn Care Res
2019
;
40
:
72
–
8
.
Holm
C
,
Hörbrand
F
,
von Donnersmarck
GH
,
Mühlbauer
W
.
Acute renal failure in severely burned patients
.
Burns
1999
;
25
:
171
–
8
.
Mosier
MJ
,
Pham
TN
,
Klein
MB
et al.
Early acute kidney injury predicts progressive renal dysfunction and higher mortality in severely burned adults
.
J Burn Care Res
2010
;
31
:
83
–
92
.
Burmeister
DM
,
Gómez
BI
,
Dubick
MA
.
Molecular mechanisms of trauma-induced acute kidney injury: inflammatory and metabolic insights from animal models
.
Biochim Biophys Acta Mol Basis Dis
2017
;
1863
:
2661
–
71
.
Simmons
EM
,
Himmelfarb
J
,
Sezer
MT
et al. ;
PICARD Study Group
.
Plasma cytokine levels predict mortality in patients with acute renal failure
.
Kidney Int
2004
;
65
:
1357
–
65
.
Cho
K
,
Pham
TN
,
Crivello
SD
,
Jeong
J
,
Green
TL
,
Greenhalgh
DG
.
Involvement of CD14 and toll-like receptor 4 in the acute phase response of serum amyloid A proteins and serum amyloid P component in the liver after burn injury
.
Shock
2004
;
21
:
144
–
50
.
Bortolin
JA
,
Quintana
HT
,
Tomé
Tde C
,
Ribeiro
FA
,
Ribeiro
DA
,
de Oliveira
F
.
Burn injury induces histopathological changes and cell proliferation in liver of rats
.
World J Hepatol
2016
;
8
:
322
–
30
.
Jeschke
MG
,
Micak
RP
,
Finnerty
CC
,
Herndon
DN
.
Changes in liver function and size after a severe thermal injury
.
Shock
2007
;
28
:
172
–
7
.
Jeschke
MG
,
Mlcak
RP
,
Finnerty
CC
et al.
Burn size determines the inflammatory and hypermetabolic response
.
Crit Care
2007
;
11
:
R90
.
Song
J
,
Finnerty
CC
,
Herndon
DN
,
Boehning
D
,
Jeschke
MG
.
Severe burn-induced endoplasmic reticulum stress and hepatic damage in mice
.
Mol Med
2009
;
15
:
316
–
20
.
Song
J
,
de Libero
J
,
Wolf
SE
.
Hepatic autophagy after severe burn in response to endoplasmic reticulum stress
.
J Surg Res
2014
;
187
:
128
–
33
.
Klein
D
,
Einspanier
R
,
Bolder
U
,
Jeschke
MG
.
Differences in the hepatic signal transcription pathway and cytokine expression between thermal injury and sepsis
.
Shock
2003
;
20
:
536
–
43
.
Barrow
RE
,
Hawkins
HK
,
Aarsland
A
et al.
Identification of factors contributing to hepatomegaly in severely burned children
.
Shock
2005
;
24
:
523
–
8
.
Gong
Y
,
Long
X
,
Xu
H
,
Yang
X
,
Guo
Q
.
The changes and prognostic value of liver function in young adults with severe burn: a retrospective observational study
.
Medicine (Baltim)
2018
;
97
:
e13721
.
Cui
L
,
Yin
S
,
Liu
W
,
Li
N
,
Zhang
W
,
Cao
Y
.
Expanded adipose-derived stem cells suppress mixed lymphocyte reaction by secretion of prostaglandin E2
.
Tissue Eng
2007
;
13
:
1185
–
95
.
Puissant
B
,
Barreau
C
,
Bourin
P
et al.
Immunomodulatory effect of human adipose tissue-derived adult stem cells: comparison with bone marrow mesenchymal stem cells
.
Br J Haematol
2005
;
129
:
118
–
29
.
François
S
,
Bensidhoum
M
,
Mouiseddine
M
et al.
Local irradiation not only induces homing of human mesenchymal stem cells at exposed sites but promotes their widespread engraftment to multiple organs: a study of their quantitative distribution after irradiation damage
.
Stem Cells
2006
;
24
:
1020
–
9
.
Toma
C
,
Wagner
WR
,
Bowry
S
,
Schwartz
A
,
Villanueva
F
.
Fate of culture-expanded mesenchymal stem cells in the microvasculature: in vivo observations of cell kinetics
.
Circ Res
2009
;
104
:
398
–
402
.
Devine
SM
,
Cobbs
C
,
Jennings
M
,
Bartholomew
A
,
Hoffman
R
.
Mesenchymal stem cells distribute to a wide range of tissues following systemic infusion into nonhuman primates
.
Blood
2003
;
101
:
2999
–
3001
.
Nesti
LJ
,
Jackson
WM
,
Shanti
RM
et al.
Differentiation potential of multipotent progenitor cells derived from war-traumatized muscle tissue
.
J Bone Joint Surg Am
2008
;
90
:
2390
–
8
.
Ra
JC
,
Shin
IS
,
Kim
SH
et al.
Safety of intravenous infusion of human adipose tissue-derived mesenchymal stem cells in animals and humans
.
Stem Cells Dev
2011
;
20
:
1297
–
308
.
Ock
SA
,
Baregundi Subbarao
R
,
Lee
YM
et al.
Comparison of immunomodulation properties of porcine mesenchymal stromal/stem cells derived from the bone marrow, adipose tissue, and dermal skin tissue
.
Stem Cells Int
2016
;
2016
:
9581350
.
Frazier
T
,
Alarcon
A
,
Wu
X
et al.
Clinical translational potential in skin wound regeneration for adipose-derived, blood-derived, and cellulose materials: cells, exosomes, and hydrogels
.
Biomolecules
2020
;
10
:
1373
.
Burmeister
DM
,
Stone
R
2nd,
Wrice
N
et al.
Delivery of allogeneic adipose stem cells in polyethylene glycol-fibrin hydrogels as an adjunct to meshed autografts after sharp debridement of deep partial thickness burns
.
Stem Cells Transl Med
2018
;
7
:
360
–
72
.
Foubert
P
,
Doyle-Eisele
M
,
Gonzalez
A
et al.
Development of a combined radiation and full thickness burn injury minipig model to study the effects of uncultured adipose-derived regenerative cell therapy in wound healing
.
Int J Radiat Biol
2017
;
93
:
340
–
50
.
Foubert
P
,
Liu
M
,
Anderson
S
et al.
Preclinical assessment of safety and efficacy of intravenous delivery of autologous adipose-derived regenerative cells (ADRCs) in the treatment of severe thermal burns using a porcine model
.
Burns
2018
;
44
:
1531
–
42
.
Ihara
K
,
Fukuda
S
,
Enkhtaivan
B
et al.
Adipose-derived stem cells attenuate pulmonary microvascular hyperpermeability after smoke inhalation
.
PLoS One
2017
;
12
:
e0185937
.
Cao
F
,
Liu
T
,
Xu
Y
,
Xu
D
,
Feng
S
.
Culture and properties of adipose-derived mesenchymal stem cells: characteristics in vitro and immunosuppression in vivo
.
Int J Clin Exp Pathol
2015
;
8
:
7694
–
709
.
Saidi
RF
,
Rajeshkumar
B
,
Shariftabrizi
A
et al.
Human adipose-derived mesenchymal stem cells attenuate liver ischemia-reperfusion injury and promote liver regeneration
.
Surgery
2014
;
156
:
1225
–
31
.
Kim
SJ
,
Park
KC
,
Lee
JU
,
Kim
KJ
,
Kim
DG
.
Therapeutic potential of adipose tissue-derived stem cells for liver failure according to the transplantation routes
.
J Korean Surg Soc
2011
;
81
:
176
–
86
.
Kim
JH
,
Park
DJ
,
Yun
JC
et al.
Human adipose tissue-derived mesenchymal stem cells protect kidneys from cisplatin nephrotoxicity in rats
.
Am J Physiol Renal Physiol
2012
;
302
:
F1141
–
50
.
Gómez
BI
,
McIntyre
MK
,
Gurney
JM
et al.
Enteral resuscitation with oral rehydration solution to reduce acute kidney injury in burn victims: Evidence from a porcine model
.
PLoS One
2018
;
13
:
e0195615
.
Burmeister
DM
,
McIntyre
MK
,
Baker
BA
et al.
Impact of isolated burns on major organs: a large animal model characterized
.
Shock
2016
;
46
:
137
–
47
.
Chao
T
,
Gómez
BI
,
Heard
TC
,
Smith
BW
,
Dubick
MA
,
Burmeister
DM
.
Burn-induced reductions in mitochondrial abundance and efficiency are more pronounced with small volumes of colloids in swine
.
Am J Physiol Cell Physiol
2019
;
317
:
C1229
–
38
.
McIntyre
MK
,
Winkler
CJ
,
Gómez
BI
et al.
The effect of burn resuscitation volumes on the gut microbiome in a swine model
.
Shock
2020
;
54
:
368
–
76
.
Gómez
BI
,
He
C
,
Chao
T
,
Dubick
MA
,
Burmeister
DM
.
Effect of intravenous fluid volumes on the adrenal glucocorticoid response after burn injury in swine
.
J Burn Care Res
2018
;
39
:
652
–
60
.
Burmeister
DM
,
Ponticorvo
A
,
Yang
B
et al.
Utility of spatial frequency domain imaging (SFDI) and laser speckle imaging (LSI) to non-invasively diagnose burn depth in a porcine model
.
Burns
2015
;
41
:
1242
–
52
.
Christy
BA
,
Herzig
MC
,
Montgomery
RK
et al.
Procoagulant activity of human mesenchymal stem cells
.
J Trauma Acute Care Surg
2017
;
83
:
S164
–
9
.
Gleeson
BM
,
Martin
K
,
Ali
MT
et al.
Bone marrow-derived mesenchymal stem cells have innate procoagulant activity and cause microvascular obstruction following intracoronary delivery: amelioration by antithrombin therapy
.
Stem Cells
2015
;
33
:
2726
–
37
.
George
MJ
,
Prabhakara
K
,
Toledano-Furman
NE
et al.
Procoagulant in vitro effects of clinical cellular therapeutics in a severely injured trauma population
.
Stem Cells Transl Med
2020
;
9
:
491
–
8
.
Tatsumi
K
,
Ohashi
K
,
Matsubara
Y
et al.
Tissue factor triggers procoagulation in transplanted mesenchymal stem cells leading to thromboembolism
.
Biochem Biophys Res Commun
2013
;
431
:
203
–
9
.
Ball
RL
,
Keyloun
JW
,
Brummel-Ziedins
K
et al.
Burn-induced coagulopathies: a comprehensive review
.
Shock
2020
;
54
:
154
–
67
.
von Bahr
L
,
Batsis
I
,
Moll
G
et al.
Analysis of tissues following mesenchymal stromal cell therapy in humans indicates limited long-term engraftment and no ectopic tissue formation
.
Stem Cells
2012
;
30
:
1575
–
8
.
Perlee
D
,
de Vos
AF
,
Scicluna
BP
et al.
Human adipose-derived mesenchymal stem cells modify lung immunity and improve antibacterial defense in pneumosepsis caused by Klebsiella pneumoniae
.
Stem Cells Transl Med
2019
;
8
:
785
–
96
.
Perlee
D
,
de Vos
AF
,
Scicluna
BP
et al.
Role of tissue factor in the procoagulant and antibacterial effects of human adipose-derived mesenchymal stem cells during pneumosepsis in mice
.
Stem Cell Res Ther
2019
;
10
:
286
.
Katsuno
T
,
Ozaki
T
,
Saka
Y
et al.
Low serum cultured adipose tissue-derived stromal cells ameliorate acute kidney injury in rats
.
Cell Transplant
2013
;
22
:
287
–
97
.
Choi
JS
,
Ryu
HA
,
Cheon
SH
,
Kim
SW
.
Human adipose derived stem cells exhibit enhanced liver regeneration in acute liver injury by controlled releasing hepatocyte growth factor
.
Cell Physiol Biochem
2019
;
52
:
935
–
50
.
Zhou
L
,
Song
Q
,
Shen
J
et al.
Comparison of human adipose stromal vascular fraction and adipose-derived mesenchymal stem cells for the attenuation of acute renal ischemia/reperfusion injury
.
Sci Rep
2017
;
7
:
44058
.
Li
J
,
Zhang
L
,
Xin
J
et al.
Immediate intraportal transplantation of human bone marrow mesenchymal stem cells prevents death from fulminant hepatic failure in pigs
.
Hepatology
2012
;
56
:
1044
–
52
.
Sang
JF
,
Shi
XL
,
Han
B
et al.
Intraportal mesenchymal stem cell transplantation prevents acute liver failure through promoting cell proliferation and inhibiting apoptosis
.
Hepatobiliary Pancreat Dis Int
2016
;
15
:
602
–
11
.
Drost
AC
,
Burleson
DG
,
Cioffi
WG
Jr,
Jordan
BS
,
Mason
AD
Jr,
Pruitt
BA
Jr.
Plasma cytokines following thermal injury and their relationship with patient mortality, burn size, and time postburn
.
J Trauma
1993
;
35
:
335
–
9
.
Mace
JE
,
Park
MS
,
Mora
AG
et al.
Differential expression of the immunoinflammatory response in trauma patients: burn vs. non-burn
.
Burns
2012
;
38
:
599
–
606
.
Dehne
MG
,
Sablotzki
A
,
Hoffmann
A
,
Mühling
J
,
Dietrich
FE
,
Hempelmann
G
.
Alterations of acute phase reaction and cytokine production in patients following severe burn injury
.
Burns
2002
;
28
:
535
–
42
.
Luz-Crawford
P
,
Djouad
F
,
Toupet
K
et al.
Mesenchymal stem cell-derived interleukin 1 receptor antagonist promotes macrophage polarization and inhibits B cell differentiation
.
Stem Cells
2016
;
34
:
483
–
92
.
Lee
K
,
Park
N
,
Jung
H
et al.
Mesenchymal stem cells ameliorate experimental arthritis via expression of interleukin-1 receptor antagonist
.
PLoS One
2018
;
13
:
e0193086
.
Amini-Nik
S
,
Dolp
R
,
Eylert
G
et al.
Stem cells derived from burned skin – the future of burn care
.
EBioMedicine
2018
;
37
:
509
–
20
.
Natesan
S
,
Wrice
NL
,
Baer
DG
,
Christy
RJ
.
Debrided skin as a source of autologous stem cells for wound repair
.
Stem Cells
2011
;
29
:
1219
–
30
.
Chan
RK
,
Zamora
DO
,
Wrice
NL
et al.
Development of a vascularized skin construct using adipose-derived stem cells from debrided burned skin
.
Stem Cells Int
2012
;
2012
:
841203
.
Published by Oxford University Press on behalf of the American Burn Association 2021. This work is written by (a) US Government employee(s) and is in the public domain in the US.
This work is written by (a) US Government employee(s) and is in the public domain in the US.